User:Rockjockey222/sandbox
Fault (Geology)
[edit]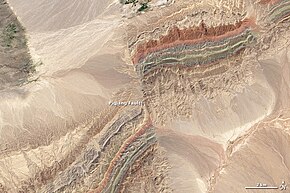
In geology, a fault is a fracture or discontinuity in a volume of rock across which there has been displacement as a result of rock-mass movement. Large faults within the Earth's lithosphere result from the motion of tectonic plates. The largest faults form at the boundaries between the plates, such as subduction zones or transform faults. The release of energy associated with rapid movement on active faults is the cause of earthquakes.[1][2]
“Fault” refers to the discontinuities mostly present in the shallow part of the earth's crust that behave in a brittle manner. At lower depths of the crust, the physical behavior of rocks change with increased pressure and temperature and deformation events occur differently. Deeper crustal deformation is usually accommodated by shear zones and the transition from faults to shear zones is known as the brittle-ductile transition zone.[2]
Fault Architecture
[edit]A fault plane is the plane that represents the fracture surface that accommodated movement during the formation of a fault. A fault trace or fault line is the two dimensional expression of a fault on the surface of the earth. A fault trace is the line commonly plotted on geologic maps to represent a fault.[1]
Because faults do not usually consist of a single fracture, geologists use the term fault zone when referring to the zone of complex deformation associated with the tabular volume of rock affected by fractures and movement. A fault zone can be centimeter to kilometer scale and is made up of a fault core and surrounding damage zone.[3] The fault core is the centralized zone of cataclastic fault rock or fault gouge and accommodated the most slip during faulting. This can be represented by a single fault plane or several fault planes with the original rock in between being intensely fractured. The damage zone is the area surrounding the fault core that is brittlely fractured above a background amount.[2][3]
Anatomy of a Fault
[edit]
The two sides of a non-vertical fault are known as the hanging wall and footwall. The hanging wall describes the volume of rock above the fault plane and the footwall occurs below it. This terminology comes from historic mining: when working a tabular ore body, the miner stood with the footwall under their feet and would hang their lantern on the hanging wall.[2][4]
Slip is defined as the amount of movement that occurs as the sides of a fault move passed each other during a deformation event. A fault's sense of slip is defined as the direction of relative motion of the rock on each side of the fault with respect to the other side.
A piercing point is a single point that was once connected and is now cut and displaced by a fault. The amount and direction of slip on the fault can be described by a displacement vector connecting the two previously adjacent points which lies in the plane or surface of the fault. This vector can be resolved into two components that describe the vertical and horizontal separation along a fault. The throw of the fault is the vertical component of the separation and the heave is the horizontal component. Any planar cross section view of the fault, which does not contain the slip vector displays an apparent offset rather than the true offset on the fault. Similarly, when a planar feature such as a sedimentary contact or a dyke margin is matched across the fault, it is usually not possible to determine the amount of offset parallel to the feature itself, so any slip estimate using a planar layer as an offset marker is also an “apparent slip”.[2]

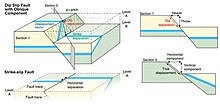
Fault Orientations
[edit]Based on direction of slip, faults can be categorized as:
- strike-slip, where the offset is predominantly horizontal.
- dip-slip, offset is predominantly parallel to the dip of the fault, and perpendicular to the fault strike.
- oblique-slip, the slip vector lies between the strike and the dip, being inclined in the plane. The angle between the slip vector and the strike line (which is horizontal), as measured within the fault plane, is described as the pitch or rake.
The orientations of faults are related to the orientation of the stress tensor and the properties of the rocks. The surface of the Earth acts as a free surface, so that two of the principal stress orientations are parallel to the surface [5][6]. This constraint prescribes the orientation of newly-formed faults as well as the conditions under which existing faults can be reactivated, which can be illustrated with the Mohr-Coulomb theory criterion.
Strike-slip faults
[edit]
In a strike-slip fault (also known as a wrench fault, tear fault, or transcurrent fault), the fault surface (plane) is usually near vertical and the footwall moves laterally either left or right with very little vertical motion[7]. Strike-slip faults with left-lateral motion are also known as sinistral faults, while those with right-lateral motion are known as dextral faults[8]. Each is defined by the direction of movement of the ground as would be seen by an observer on the opposite side of the fault.
A special class of strike-slip fault is the transform fault, and can be found at plate boundaries. These faults are created from the offsets caused by plate rate discontinuities across spreading centers known as a mid-ocean ridges, but can also be found within the continental lithosphere, such as the Dead Sea Transform in the Middle East or the Alpine Fault in New Zealand. Transform faults are also referred to as "conservative" plate boundaries, because lithosphere is neither created nor destroyed[9].
Dip-slip faults
[edit]Dip-slip faults can be either normal ("extensional") or reverse (“shortening”). The terminology of "normal" and "reverse" comes from coal-mining in England, where normal faults are the most common[10].
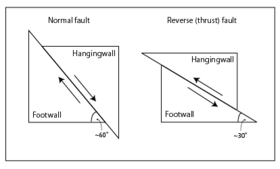
In a normal fault, the hanging wall moves downward relative to the footwall. Normal faults typically occur in extensional environments, where the landmass on either side of a fault pulls apart. The dip of a normal fault is typically 60-70° near the surface of the earth in an extensional stress regime, but often changes with depth (see section Special Geometries). A downthrown block between two normal faults dipping towards each other is a graben. An upthrown block between two normal faults dipping away from each other is a horst. Low-angle normal faults with regional tectonic significance may be designated as as detachment faults.
A thrust or reverse fault is the opposite of a normal fault, where the hanging wall moves upwards relative to the footwall. These faults indicate shortening of the crust, where two blocks are pushed towards one another. The fault is considered a thrust fault at dip angles of 10-30°[2], but at steeper dips the term reverse fault is used instead.
Faults may be reactivated at a later time with movement in the opposite direction to the original slip. This is known as fault inversion, and can result in a normal fault becoming a reverse fault or vice versa[11].
Oblique-slip faults
[edit]
A fault which has components of dip-slip and strike-slip is considered an oblique-slip fault. Nearly all faults will have some component of both dip-slip and strike-slip, so defining a fault as oblique requires both dip and strike components to be measurable and significant. Oblique faults can occur within transtensional or transpressional regimes, while others form when the direction of extension or shortening changes during deformation with pre-existing faults remaining active[2].
Special Geometries
[edit]Listric fault
[edit]
Listric faults are similar to normal faults but the fault plane curves, with the dip being steeper near the surface, then shallower with increasing depth. The dip may flatten into a nearly horizontal décollement, resulting in horizontal slip[12]. The illustration shows slumping of the hanging wall along a listric fault.
Ring fault
[edit]Ring faults, also known as caldera faults, are faults that occur within collapsed volcanic calderas and the sites of bolide strikes, such as the Chesapeake Bay impact crater[13]. Ring faults are the result of a series of overlapping normal faults, forming a circular outline. Fractures created by ring faults may be filled by ring dikes.
Flats and ramps
[edit]
Flat segments of thrust fault planes are known as flats, and inclined sections of the thrust are known as ramps. Typically, thrust faults move within formations by forming flats and climb up sections with ramps. Thrust faults form nappes and klippen in the large thrust belts. Subduction zones are a special class of thrusts that form the largest faults on Earth and give rise to the largest earthquakes.
Fault-bend folds are formed by movement of the hanging wall over a non-planar fault surface and are found associated with both extensional and thrust faults.
Synthetic and antithetic faults
[edit]Synthetic and antithetic faults are terms used to describe minor faults associated with a major fault[14]. Synthetic faults dip in the same direction as the major fault while antithetic faults dip in the opposite direction. These faults may be accompanied by rollover anticlines (e.g. the Niger Delta Structural Style).
Fault Rocks
[edit]The most commonly used terms are based off of the amount of matrix interstitial to the clasts left over after the breaking down of the host rock, the amount of clasts, the size of the clasts present, and whether or not the rock is cohesive.[15][2][16]
The main types of brittle fault rocks are:
- Cataclasite – a cohesive, granular fault rock with a low fragment to matrix ratio.
- Fault Breccia – an unconsolidated fault rock with less than 30% matrix.
- Fault Gouge – a fault rock with more than 30% matrix.
- Pseudotachylyte – microcrystalline or glass, quenched frictional melts. Chilled margins, injection veins, and fragments of host rock are often observed.
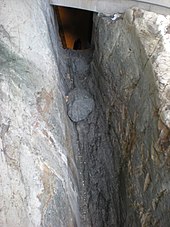
Fault Mechanisms
[edit]Abrasive wearing is recognized as the dominant deformation mechanism for brittle fault rocks above the brittle ductile-transition zone, although fault rocks an sometimes have a foliation.[17]
Active Faults and Seismic Hazard
[edit]Assessing the threat of potential earthquakes posed by active faults, and trying to mitigate the destruction or losses of life that they can impose, is the main driving force for seismologists in the study of faults[18]. Active faults can be found across the world, but are most commonly found along plate boundaries. The size of an earthquake is directly linked to the area of a rupture surface and the distance in which it slips during an event. Earthquake ruptures can vary from the microscopic scale to many hundreds of kilometres in length, with the largest event ever recorded occurring in Valdivia Chile in 1960. The magnitude of this event was Mw 9.5, and the length of the fault rupture was a staggering 800 km[19].
Fault-Related Hazard
[edit]Seismic hazard is a measure of the probability of a damaging earthquake occurring in an area over a given time length, typically on the scale of decades[20]. Cities or important structures (such as pipelines, mines, or bridges) in areas with a significant risk of seismicity are often required to be built to a higher standard, in which they can withstand the maximum predicted shaking a region may experience. There is a large discrepancy in earthquake-related casualties based on the building quality and population density of any given country[21]. Earthquakes can also cause far more damage if located close to the surface, as the energy is less dissipated by the time it reaches a population center.
In geotechnical engineering a fault often forms a discontinuity that may have a large influence on the mechanical behavior (strength, deformation, etc.) of soil and rock masses in, for example, tunnel, foundation, or slope construction. In California, for example, new building construction has been prohibited directly on or near faults that have moved within the Holocene Epoch (the last 11,700 years) of the Earth's geological history.[22] Also, faults that have shown movement during the Holocene plus Pleistocene Epochs (the last 2.6 million years) may receive consideration, especially for critical structures such as power plants, dams, hospitals, and schools. Geologists assess a fault's age by studying soil features seen in shallow excavations and geomorphology seen in aerial photographs. Subsurface clues include shears and their relationships to carbonate nodules, eroded clay, and iron oxide mineralization, in the case of older soil, and lack of such signs in the case of younger soil. Radiocarbon dating of organic material buried next to or over a fault shear is often critical in distinguishing active from inactive faults. From such relationships, paleoseismologists can estimate the sizes of past earthquakes over the past several hundred years, and develop rough projections of future fault activity.
Associated Natural Disasters
[edit]Another side effect of large earthquakes is the potential for tsunamis, which can cause destruction rivaling or exceeding that of the earthquake itself. Tsunamis are generated by the displacement of a large amount of water due to the slip of a rupture surface underwater[23].This was seen following the Tohoku earthquake in Japan in 2011[24]. The intense shaking from large earthquakes can also trigger landslides. This occurred following the Denali fault rupture in 2002, which caused landslides at distances exceeding hundreds of kilometers from the earthquake source[25].
Economic Implications
[edit]The presence of subsurface faults is significant to the mining and oil industries because they can be linked to ore bodies or as a petroleum trap. Some fault types can act as effective seals, preventing the flow of oil from leaving a reservoir rock[26]. This then results in an oil-saturated medium which can be extracted by conventional methods. Faults can also act as a fluid conduit, and in the case of epithermal systems, can transport minerals in solution until they precipitate out due to a drop in temperature[27]. This can result in the concentration of ore, and these fault zones can become economically viable for mining.
Impacts on structures and people
[edit]See also
[edit]References
[edit]- ^ a b "Earthquake Glossary". earthquake.usgs.gov. Retrieved 2019-12-11.
- ^ a b c d e f g h Fossen, Haakon, 1961-. Structural geology (Second edition ed.). Cambridge, United Kingdom. ISBN 978-1-107-05764-7. OCLC 946008550.
{{cite book}}
:|edition=
has extra text (help)CS1 maint: multiple names: authors list (link) CS1 maint: numeric names: authors list (link) - ^ a b Chester, F. M.; Logan, J. M. (1986). "Implications for mechanical properties of brittle faults from observations of the Punchbowl fault zone, California". Pure and Applied Geophysics PAGEOPH. 124 (1–2): 79–106. doi:10.1007/BF00875720. ISSN 0033-4553.
- ^ Tingley, Joseph V. (2000). Traveling America's loneliest road : a geologic and natural history tour through Nevada along U.S. Highway 50. Pizarro, Kris Ann. (1st ed ed.). Reno: Nevada Bureau of Mines and Geology. ISBN 1-888035-05-6. OCLC 44159942.
{{cite book}}
:|edition=
has extra text (help) - ^ Anderson, E. M. (1951). The dynamics of faulting and dyke formation with applications to Britain. Hafner Pub. Co.
- ^ Coulomb, C. A. (1776). "Essai sur une application des regles des maximis et minimis a quelquels problemesde statique relatifs, a la architecture". Mem. Acad. Roy. Div. Sav. vol. 7: 343-387.
{{cite journal}}
:|volume=
has extra text (help) - ^ Allaby, Michael (2013). A Dictionary of Geology and Earth Sciences. Oxford University Press. ISBN 978-0-19-965306-5.
- ^ Park 2004
- ^ Tarling, D. H. (1983). Orogenic Andesites and Plate Tectonics J. B. Gill, Springer-Verlag, Berlin, 1981 390 pp., 27.95 (73(2) ed.). Geophysical Journal International. ISBN 10.1093/gji/73.2.581-a.
{{cite book}}
: Check|isbn=
value: invalid character (help) - ^ Peacock D.C.P.; Knipe R.J.; Sanderson D.J. (2000). "Glossary of normal faults". Journal of Structural Geology. 22 (3): 298. doi:10.1016/S0191-8141(00)80102-9.
- ^ Sibson, Richard H (January 1985). "A note on fault reactivation". Journal of Structural Geology. 7 (6): 751–754. doi:https://doi.org/10.1016/0191-8141(85)90150-6.
{{cite journal}}
: Check|doi=
value (help); External link in
(help)|doi=
- ^ McClay, K. R.; Ellis, P. G. (1987). "Analogue models of extensional fault geometries". Geological Society, London, Special Publications. 28 (1): 109–125. doi:https://doi.org/10.1144/GSL.SP.1987.028.01.09.
{{cite journal}}
: Check|doi=
value (help); External link in
(help)|doi=
- ^ Poag, C. Wylie; Koeberl, Christian; Reimold, Wolf Uwe (2004). The Chesapeake Bay Crater: Geology and geophysics of a Late Eocene submarine impact structure.
- ^ Peacock, D. C. P; Sanderson, D. J (1 January 1991). "Displacements, segment linkage and relay ramps in normal fault zones". Journal of Structural Geology. 13 (6): 721–733. doi:10.1016/0191-8141(91)90033-F. ISSN 0191-8141.
- ^ SIBSON, R. H. (1977-03). "Fault rocks and fault mechanisms". Journal of the Geological Society. 133 (3): 191–213. doi:10.1144/gsjgs.133.3.0191. ISSN 0016-7649.
{{cite journal}}
: Check date values in:|date=
(help) - ^ Woodcock, N. H.; Mort, K. (2008-05). "Classification of fault breccias and related fault rocks". Geological Magazine. 145 (3): 435–440. doi:10.1017/S0016756808004883. ISSN 0016-7568.
{{cite journal}}
: Check date values in:|date=
(help) - ^ Bestmann, M.; Pennacchioni, G.; Nielsen, S.; Göken, M.; de Wall, H. (2012-05-01). "Deformation and ultrafine dynamic recrystallization of quartz in pseudotachylyte-bearing brittle faults: A matter of a few seconds". Journal of Structural Geology. Physico-Chemical Processes in Seismic Faults. 38: 21–38. doi:10.1016/j.jsg.2011.10.001. ISSN 0191-8141.
- ^ Lay, Thorne. (1995). Modern global seismology. Wallace, Terry C. San Diego: Academic Press. ISBN 978-0-08-053671-2. OCLC 176630610.
- ^ Barrientos, Sergio E.; Ward, Steven N. (December 1990). "The 1960 Chile earthquake: inversion for slip distribution from surface deformation". Geophysical Journal International. 103 (3): 589–598. doi:https://doi.org/10.1111/j.1365-246X.1990.tb05673.x.
{{cite journal}}
: Check|doi=
value (help); External link in
(help)|doi=
- ^ McGuire, Robin (October 1996). "Probabilistic seismic hazard analysis and design earthquakes: closing the loop". International Journal of Rock Mechanics and Mining Sciences & Geomechanics Abstracts. 33 (7): A294. doi:10.1016/0148-9062(96)83355-9.
- ^ Holzer, Thomas L.; Savage, James C. (February 2013). "Global Earthquake Fatalities and Population". Earthquake Spectra. 29 (1): 155–175. doi:https://doi.org/10.1193/1.4000106.
{{cite journal}}
: Check|doi=
value (help); External link in
(help)|doi=
- ^ Brodie et al. 2007
- ^ "How do earthquakes generate tsunamis?". University of Washington. Archived from the original on 2007-02-03.
- ^ Mori, Nobuhito; Takahashi, Tomoyuki; Yasuda, Tomohiro; Yanagisawa, Hideaki (2011). "Survey of 2011 Tohoku earthquake tsunami inundation and run-up". Geophysical Research Letters. 38 (7). doi:10.1029/2011GL049210. ISSN 1944-8007.
- ^ Jibson, Randall W.; Harp, Edwin L.; Schulz, William; Keefer, David K. (August 2004). "Landslides Triggered by the 2002 Denali Fault, Alaska, Earthquake and the Inferred Nature of the Strong Shaking". Earthquake Spectra. 20 (3): 669–691. doi:https://doi.org/10.1193/1.1778173.
{{cite journal}}
: Check|doi=
value (help); External link in
(help)|doi=
- ^ Watts, N.L. (November 1987). "Theoretical aspects of cap-rock and fault seals for single- and two-phase hydrocarbon columns". Marine and Petroleum Geology. 4 (4): 274–307. doi:https://doi.org/10.1016/0264-8172(87)90008-0.
{{cite journal}}
: Check|doi=
value (help); External link in
(help)|doi=
- ^ SIBSON, R. H.; MOORE, J. Mc. M.; RANKIN, A. H. (November 1975). "Seismic pumping—a hydrothermal fluid transport mechanism". Journal of the Geological Society. 131 (6): 653–659. doi:https://doi.org/10.1144/gsjgs.131.6.0653.
{{cite journal}}
: Check|doi=
value (help); External link in
(help)|doi=
![]() | This is a user sandbox of Rockjockey222. You can use it for testing or practicing edits. This is not the sandbox where you should draft your assigned article for a dashboard.wikiedu.org course. To find the right sandbox for your assignment, visit your Dashboard course page and follow the Sandbox Draft link for your assigned article in the My Articles section. |