User:Laurenferruccio/sandbox
Brain Energy Metabolism and Psychiatric Disorders
[edit]By:
Lauren Ferruccio
Nikola Ivetic
Sarah Lal
Biochem 3D03 "Your Way" project.
Introduction
[edit]The origins and causes of numerous psychiatric disorders in the mind is a large and booming area of investigation. Not much is known on what exactly causes psychiatric disorders to develop, but throughout the years a large growing pattern began to emerge, linking metabolic processes to psychiatric disorders.[1] Early researchers quickly identified the fact that symptoms involving hypoxemia often included mental deficiencies such as loss of judgment or memory, time disorientation, irritability, emotional instability, and overall irrational behavior, traits which are common in psychiatric disorders.[1] In the brain, a close correlation was determined linking local brain activity with that of the region's local glucose metabolism rate; these findings suggested a tight coupling between energy substrate production and regional brain activity. Overall it has been shown that memory, cognition and neuronal cell survival all depend on optimum glucose use and energy production within the brain.[2] Deficiencies in these basic energy pathways has been linked to numerous brain disorders(Table 1).[1] Given the relativity large size and complexity of the human brain and the overall huge variation in mental disorders, a general focus on metabolism in schizophrenia and depression, as well as ways of monitoring brain energy metabolism, are assessed.
Brain metabolism in normal subjects
[edit]The brain is a complex, poorly understood organ. It is known to account for approximately 20% of the body’s total glucose requirements, hypothesized to be due to the extreme energy requirements of the sodium-potassium pump, which forms the basis for the majority of the brain’s functions.[3] Glucose is also indirectly used for the biosynthesis of neurotransmitters, which are important signaling molecules that are often responsible for activating or deactivating sodium-potassium pumps, or binding to other receptors. As a multifunctional organ, the brain contains numerous cell types, each of which carries out a specific function.
Metabolism in the brain is similar to metabolism elsewhere in the body. Glucose is the main substrate for energy production, typically used at a rate of 31mmol per 100g of wet weight every minute.[4] Of this, 87% is used for oxidative phosphorylation, while the remaining 13% is used for other processes, most notably the synthesis of neurotransmitters. Lactate and pyruvate can also be used for oxidative phosphorylation, but since they cannot cross the blood-brain barrier, they must be synthesized within the brain.
Glycolysis
[edit]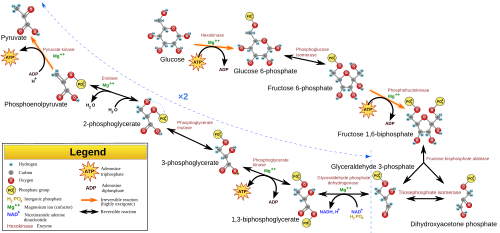
Glucose enters the brain via specialized glucose transporter proteins, where it then enters various cell types, particularly astrocytes and neurons. [3][4] Astrocytes are heavily involved in metabolism, reuptake of neurotransmitters, and potassium homeostasis. They have also been found to use the majority of the glucose within the brain.
When the brain is activated, glucose tends to enter the astrocytes, where it undergoes glycolysis to form both lactate and pyruvate (Figure 1).[3] The first step in glycolysis, catalyzed by hexokinase, requires an input of energy in the form of adenosine-5’-triphosphate (ATP). Within astrocytes, hexokinase binds to the outer mitochondrial membrane via a porin protein. This results in a preferential use of ATP generated by the mitochondria through oxidative phosphorylation, as opposed to glycolytically-derived ATP. This process also occurs in muscle cells, but it is much less common. After glycolysis is complete, pyruvate is formed, which can also be converted into lactate. Much of the pyruvate continues on to the tricarboxylic acid (TCA) cycle (Figure 2) and electron-transport chain (ETC), as astrocytes are the primary cell type in which these processes occur in the brain. Lactate and pyruvate can also be released from astrocytes to be used by neurons, through the monocarboxylic acid transporter (MCT), however uptake of these molecules tends to be fairly slow. Astrocytes also contain the brain’s only glycogen reserve, which is thought to act as a fast energy source to bridge the gap between initiation of brain activity and increased delivery of free glucose to the brain.
Neurons can also take up glucose directly, particularly in the white matter where astrocytes are much less abundant.[3] In white matter, oligodendrocytes make up the majority of cells, but they have extremely low rates of glucose metabolism and utilization, instead relying largely on what is produced by the neurons.
The Tricarboxylic Acid Cycle and Neurotransmitter Biosynthesis
[edit]
A key metabolic intermediate within the cell is acetyl-CoA. In the brain, this is primarily derived from pyruvate, but it can also be formed from fatty acids (in astrocytes), ketone bodies (neurons, astrocytes and oligodendrocytes) and monocarboxylic acids such as lactate.[4] Once acetyl-CoA is formed, there are many possible fates. It is a biosynthetic precursor to the neurotransmitter acetylcholine, and also feeds into the TCA cycle.
The TCA cycle has multiple purposes. The most common purpose is to produce NADH and FADH2 for oxidative phosphorylation, but several key intermediates within the TCA cycle are particularly important for cataplerosis. [3] Within the context of the brain, these cataplerotic reactions are primarily involved in neurotransmitter biosynthesis (Table 2):
Table 2: Biosynthetic precursors of key neurotransmitters. [3]
Neurotransmitter | Biosynthetic precursor(s) |
---|---|
Acetylcholine | Citrate, acetyl-CoA |
Glutamine | Glutamate (astrocytes and glial cells only) |
Glutamate | α-ketoglutarate |
γ-amino butyric acid (GABA) | Glutamate (by PLP-dependent glutamate decarboxylase) |
Serine | 3-phosphoglycerate |
Glycine | Serine |
Alanine | Pyruvate (via transamination) |
Aspartate | Oxaloacetate |
Due to the cataplerosis necessary to maintain brain function, anaplerosis is also necessary in order to maintain the TCA cycle.[3] The most important reaction in this regard is catalyzed by pyruvate carboxylase, which carboxylates pyruvate to form oxaloacetate. This enzyme is only present in astrocytes, not neurons, hence astrocytes are the main site of neurotransmitter biosynthesis; they are the only cell type capable of compensating for the cataplerosis with anaplerosis. Additional neurotransmitters such as dopamine, epinephrine and norepinephrine are biosynthesized from aromatic amino acids, thus indirectly contributing to the anaplerosis that occurs.
The Role of Neurotransmitters and the Psychosomatic Continuum
[edit]Neurotransmitters are very important regulators within the brain, though many of their functions are not fully understood. They originally evolved solely to regulate energy metabolism, but have expanded to include regulation of growth signals, the sympathetic and parasympathetic nervous systems, cerebral blood flow in the brain, and intercellular communication, which involves both affective and cognitive processes.[3] As a result of this, many stresses caused to the affective and cognitive processes can cause metabolic stress, and vice versa. This is termed the “psychosomatic continuum”. For example, acute stress is known to activate the cholinergic system (the “fight or flight” response), but chronic stress causes cholinergic degradation. This results in behavioural changes, such as the learning deficits caused by chronic stress and the subsequent cholinergic degradation.
Regulation of Energy Metabolism
[edit]Energy metabolism in the brain has additional regulation checkpoints not seen in other cell types. It is important to note that astrocytes, the main cell type involved in brain energy metabolism are non-excitable, and thus cannot directly respond to the glutamatergic impulses that cause excitation of neuron cells.[3] However, excitation results in increased sodium-potassium pumping, causing an increase in potassium ion concentrations. In astrocytes, metabolism is upregulated by a potassium-sensitive site on the ATPase that senses increases in potassium and glutamate levels. When this occurs, glycolysis, glycogenolysis and the TCA cycle are all activated in astrocytes, due to a simultaneous need for energy as well as excitatory amino acids during periods of neuronal excitation. In neurons, excitation is the major stimulant for glucose metabolism. There is also much crosstalk between neurons and astrocytes, as shown in Figure 3.

Energy metabolism under special conditions
[edit]Although glucose is the preferred energy source for the brain, certain conditions can require the use of alternative sources. Such conditions include starvation (both chronic and acute), patients with diabetes, and newborns being breastfed.[4] In these cases, ketone bodies are used as energy sources, particularly acetoacetate and D-3-hydroxybutyrate. Astrocytes are particularly important for this as well, since they have the ability to produce ketone bodies in a similar manner as hepatocytes. Under these conditions, gluconeogenesis can also occur within the astrocytes, but this is not typical under normal circumstances.[3]
Diagnosis and Treatment of Abnormalities in Brain Metabolism
[edit]A significant impact of alterations in brain metabolism and brain chemistry is that these may ultimately manifest themselves in the form of psychiatric disorders in the affected individual. Such disorders are usually attributed to depressive or psychotic symptoms that are self-reported, reported by another individual, or observed by a medical professional. Furthermore, the diagnosis of an individual with an affective disorder, such as depression, may be highly subjective and greatly influenced by the past experiences and the knowledge possessed by the physician. There are many cases of under- and over-diagnoses in existence.
Depression
[edit]Using [18F]-FDG tracers and PET studies, researchers noted that patients who suffer from bipolar depression exhibited lower brain metabolic rates in comparison to that of control groups. Interestingly, the metabolic rates within the entire brain increased when subjects shifted from a state of depression into that of a euthymic or manic state. Unipolar depressed subjects exhibited significantly lower metabolic rates in the lower caudate nucleous region of the brain then even that of bipolar tested subjects. All of these results were gleaned from the use of [18F]-FDG, which directly shows where glucose is occurring and is discussed further below. [8]. When researchers performed the [11C]-glucose tests, done to show where the concentration of amino acids were found. They showed that bipolar manic patients slowed a globally increased level of cortical amino acid pools, while unipolar depressed subjects had a reduced level of amino acids. These results suggest that a disturbance is present in the amount of amino acids present in the cortical brain regions of unipolar and bipolar manic patients. [8] Overall the results indicated that depressed subjects have a reduced glucose metabolic rate when compared to controls.
Schizophrenia
[edit]Early studies involving people who suffer from psychiatric disorders (especially schizophrenics), showed that they possessed an abnormal disturbance in glucose metabolism. In particular patients with chronic psychiatric disorders displayed sustained hyperglycemia, prolonged hyperinsulinemia and elevated lactic acid levels in the blood following glucose tolerance testing. [2] Overall schizophrenics were found to also have a significant predisposition for developing Type II diabetes (15.8% vs 2-3% chance from the general population). More modern proteomic, metabolomic and genomic studies have shown that schizophrenics in particular develop an altered brain energy profile consistent with that of a state of hypoxia within the prefrontal cortex. Analyzing the prefrontal cortex using the systems analysis approach (transcriptomic, proteomic, and metabolomic comparisons) indicated that the glycolysis pathway was significantly downregulated in schizophrenics. Expression levels of four glycolytic genes were lower than normal, and proteomic results showed that 7 of the 10 glycolyic proteins were downregulated. Pyruvate dehydrogenase (PDH) levels were significantly lowered in protein amount(along with reduced transcription levels of 2 of its subunits). This PDH reduction corresponds to an increase in lactic acid accumulation and cellular acidosis in schizophrenics. These results taken together indicated a shift towards anaerobic respiration in the brain.[9] In addition to this change, isoforms of the glycogen catabolism 1,4-debranching enzyme as well as the glucose-6-phosphate transporter were up-regulated in schizophrenics. This coupled with a marked decrease in expression of glycogen synthesis enzymes demonstrates an increase in glycogen catabolism within the schizophrenic mind, indicating an increased demand for glucose. [9]
Treatments
[edit]Upon diagnosis, methods used to treat such psychiatric ailments vary greatly in accordance to what is most suitable for the individual afflicted by the disorder. For depression in particular, these forms of treatment include the use of drugs with antidepressant properties, psychotherapy and, in extreme cases wherein the first two types of treatment are not effective, electroconvulsive therapy (ECT). The ultimate goal of all these treatments is for the patient to attain complete relief from the symptoms of depression. In particular, antidepressant drugs exhibit their effects through altering brain chemistry, especially in how neurotransmitters such as norepinephrine and serotonin work in the brain. Psychotherapy aims to address the underlying issues causing depression in an individual, whilst electroconvulsive therapy works through inducing a short seizure in an anaesthetized individual using electric current. The exact mechanism of how ECT works is unknown.
Although the diagnosis of depression and other affective disorders on the basis of observations of external symptoms is prone to a high degree of subjectivity, the functional and structural changes that are often present in individuals affected by these disorders are not. It is often useful to monitor the efficacy of patient treatment, whether using antidepressants, psychotherapy, ECT or other methods, through observation of structural and functional changes in the brain as it, optimally reverts back to that which is observed in a healthy patient. For this application, the use of brain imaging tools is extremely useful. The following section discusses several brain imaging techniques used for three different scopes: monitoring changes in brain activity levels, monitoring structural changes in the brain, and monitoring changes in brain function during brain stimulation treatments.
Brain Imaging
[edit]Monitoring Changes in Brain Function
[edit]There are several useful techniques to monitor changes in brain function, many of which can be used to gain insight into how energy metabolism is working as well.
Positron Emission Tomography (PET)
[edit]How does it work?
Compounds that have been radiolabelled with positron-emitting radionuclides are injected into the bloodstream.[10] Their accumulation in select regions of the brain is indicative of neurotransmitter activities, glucose metabolism, and other brain activities. Radiolabelling provides a means by which to trace these compounds in the brain through detection of gamma rays emitted by the radionuclide.
Pathways and/or Metabolites Monitored
1. Changes in glucose metabolism (using [18F]-FDG):[11][12] This radioligand is taken up by the most energy demanding cells, including the brain, tumors, and kidneys, as shown in Figure 4:
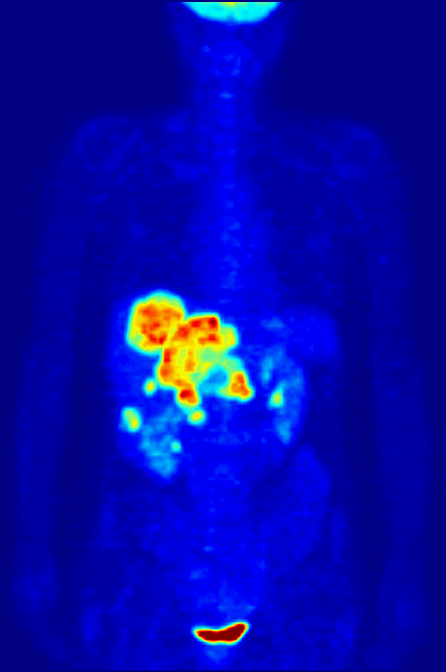
2. Dopamine receptor activity (using [11C]NNC-112)[14] and [11C]Raclopride:[15][16] [11C]-NNC-112 and [11C]-raclopride are dopamine analogues and very useful PET radioligand for the observation of functional activity of striatal and neocortical dopamine receptors.
3. Serotonin transporters (using [11C]DASB):[15] This radioligand exhibits extremely high binding to serotonin transporters in the thalamus, striatum and mid-brain and lower binding in other areas of the brain.
4. L-amino decarboxylase enzyme activity (using [18F]FDOPA):[17] [18][19]or [11C]DOPA[20] As L-DOPA analogues, these compounds are metabolized as such; they are biological precursors to catecholamine hormones such as norepinephrine and dopamine.
Normal vs. Abnormal Function of the Pathway
1. Decreased glucose metabolism observed using [18F]-FDG PET in patients with depression[12] and those with schizophrenia.[11]
2. Higher binding of [11C]-raclopride(D) and [11C]NNC-112[14] to dopamine receptors in depression due to a lower amount of endogenous dopamine. No changes in dopamine levels in schizophrenia were observed using [11C]raclopride.[16]
3. Decreased uptake of [11C]DASB using serotonin receptors in depression.[15]
4. High accumulation of [18F]-FDOPA or [11C]DOPA in patients with schizophrenia, indicating a high production of endogenous dopamine. Low accumulation in patients with depression, consistent with low production of dopamine.[17][19][18][19][20]
Results for Healthy Individuals vs. Individuals with Affective Disorders
Single Photon Emission Computed Tomography (SPECT)
[edit]How does it work?
Gamma-emitting radionuclide-labeled compounds or free radionuclides are injected into a patient.[10] Their accumulation in select regions of the brain is indicative of neurotransmitter activities, glucose metabolism, and other brain activities. Radiolabelling provides a means by which to trace these compounds in the brain through detection of gamma rays emitted by the radionuclide.The chemical characteristics of the injected compound will cause it to aggregate in a known region of the brain, or it will be metabolized through a known process. Variations in terms of localization of the molecule or its metabolism are generally associated with abnormalities in brain chemistry or metabolic processes.
Pathways and/or Metabolites Monitored
1. Serotonin transporters (using [123I]-ADAM, which binds selectively to these transporters).[21]
Note: The number of radionuclides amenable to SPECT is limited, especially when compared to PET imaging.
Normal vs. Abnormal Function of the Pathway
1. Decreased binding of [123I]-ADAM to serotonin receptors in depression[21] and increased serotonergic activity in schizophrenia. [22]
Results for Healthy Individuals vs. Individuals with Affective Disorders
Source: http://imaging.ubmmedica.com/CME/pt/content/2007/0712/figure_bransfield.gif
Electroencephalography (EEG)
[edit]How does it work?
Electrodes are placed on either side of the scalp for the purpose of detecting brain electrical activity. The electrical output of the brain can be measured in all parts of the brain simultaneously, and the position or time of their occurrence may be determined precisely. Due to poor resolution and a desire to visualize the brain, however, EEG is often combined with other methods of imaging.
Pathways and/or Metabolites Monitored
Brain metabolic pathways and/or metabolites being influenced by the affective disorder may be implicated from the results of the scan. For this reason, EEG scans are often used in combination with other brain imaging methods. This way, more information about any abnormalities in brain function can be elucidated and correlated with specific affective disorders, if applicable.
Normal vs. Abnormal Function of the Pathway
Scans are compared with averaged scans of individuals who do not possess any signs or symptoms of affective disorders. Note: EEG scans are rarely used as stand-alone methods and, as such, are generally combined with other imaging methods to provide more information with regards to brain function.
Results for Healthy Individuals vs. Individuals with Affective Disorders
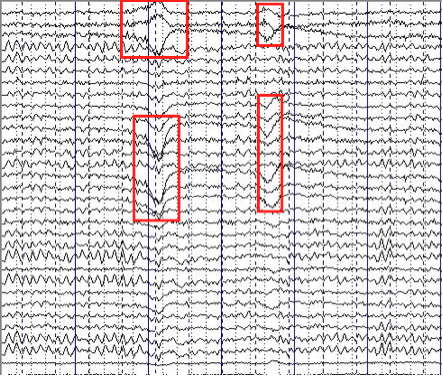
Source: http://www.cerebromente.org.br/n03/tecnologia/eeg.htm
Functional Magnetic Resonance Imaging (fMRI)
[edit]How does it work?
Through the use of a strong magnetic field, nuclei in the region of the brain of interest are aligned and then achieve higher levels of magnetization. [10] Their accumulation in select regions of the brain is indicative of neurotransmitter activities, glucose metabolism, and other brain activities. Radiolabelling provides a means by which to trace these compounds in the brain through detection of gamma rays emitted by the radionuclide. Upon removing the magnetic field, the nuclei emit energy, which is dependent on their location in the brain, and this energy is measured and related to their positions in the brain.
The differing magnetic properties of arterial vs. venous blood are exploited in fMRI. In particular, deoxyhemoglobin is paramagnetic and serves as an endogenous contrast agent and is the source of signal in fMRI. Neural activity is generally accompanied by increases in blood used, to provide oxygen via hemoglobin, in that area, which in turn gives rise to reductions in deoxyhemoglobin that can be observed as functional brain activity. [23]
Pathways and/or Metabolites Monitored
Brain metabolic pathways and/or metabolites being influenced by the affective disorder may be implicated from the results of the scan. fMRI is usually combined with other imaging methods to provide further information on brain function (EEG) or used in combination with stimulation-based therapies (such as Transcranial Magnetic Stimulation, TMS) to monitor improvements in brain function during successive treatments.
Normal vs. Abnormal Function of the Pathway
fMRI scans are generally used to monitor changes in brain function in the individual before, during and after treatment of disorders affecting the brain.
Results for Healthy Individuals vs. Individuals with Affective Disorders
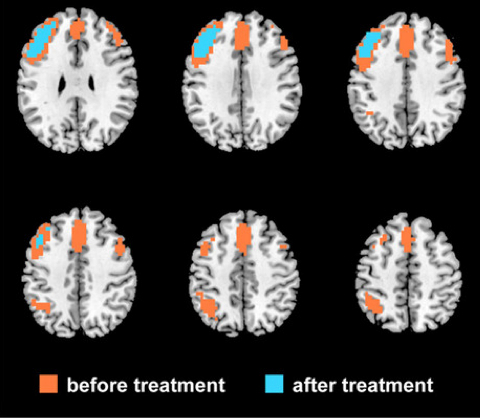
Source: Perrin, J.S., et al. (2012) Electroconvulsive therapy reduces frontal cortical connectivity in severe depressive disorder. PNAS (Early Edition; available online only on 2012/03/22). doi: 10.1073/pnas.1117206109
Monitoring Structural Changes in the Brain
[edit]Magnetic Resonance Imaging (MRI)
[edit]How does it work?
Through the use of magnetic fields and radio waves, it is possible to produce 2D images (or 3D images through compilation of 2D images) of structures inside the brain.[10] Their accumulation in select regions of the brain is indicative of neurotransmitter activities, glucose metabolism, and other brain activities. Radiolabelling provides a means by which to trace these compounds in the brain through detection of gamma rays emitted by the radionuclide. Such images may be obtained without the administration of a radiolabelled compound. A large cylinder-shaped magnet that surrounds the patient’s head is used; radio waves are transmitted through the head and, in the presence of the magnetic field, different parts of the brain will produce different frequencies that allows for the construction of an image. Both surface or subsurface, and cross-sectional details of the brain may be obtained. Note that no information on brain function is obtained through MRI; supplementary information on brain function and any abnormalities must be obtained through complementary methods such as those previously mentioned.
Results for Healthy Individuals vs. Individuals with Affective Disorders
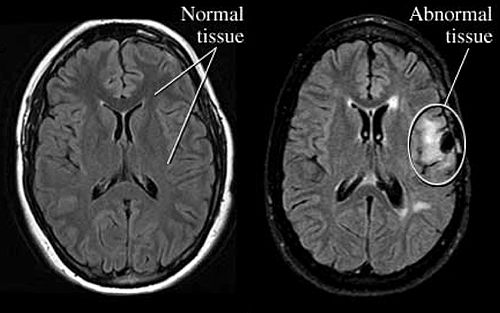
Source: https://myhealth.alberta.ca/health/_layouts/healthwise/media/medical/hw/h9991111.jpg
Note: All aforementioned methods are often used in combination with other methods of detection. For example, structural information about the brain is often supplemented with information on its functional activity due to the fact that alternations in brain structure itself are not directly correlated with changes in brain function to illicit the onset of affective disorders; for this to be determined, methods that obtain information on brain functional activity must be used.
Monitoring Changes in Brain Function During Brain Stimulation Treatments
[edit]Functional and structural imaging techniques are often useful to monitor progress of patients with affective disorders before, during and after treatment with transcranial magnetic stimulation and electroconvulsive therapy.
The effect of drugs on brain energy metabolism
[edit]Interference with dopamine signaling remains the key primary target of action with anti-psychotic drugs. However, interestingly enough was that research indicated anti-psychotic drugs also played some influence in glucose metabolism. Common drugs such as chlorpromazine, fluphenazine, and thioridazine all generated hypoglycemia within test subjects, some of which furthermore also developed symptoms of diabetes. Recent studies have actually determined that many anti-psychotic drugs inhibit glucose transport into neuronal cell types. Though the actual method of how these drugs accomplish this role is unknown, recent results have pointed towards the drugs actually being able to bind and block glucose transport proteins directly. This results in a direct global depression of glucose metabolism as measured by [18F]-FDG). Despite all these observations, it is still not precisely clear on whether glucose uptake inhibition contributes appreciably to the therapeutic benefits of the drugs, or whether it causes adverse effects. [2]
Case Study
[edit]Profile: Paul is a 45 year old chemistry teacher at Pearl Island High School. He is extremely ambitious and passionate about his work, however lately he has been taking a lot of sick days. Colleagues claim that he is not as sociable or “on top of things” as he used to be. Furthermore, he is no longer participating in sports he once found enjoyable and his children complain that he is irritable and never spends time with them like he used to. He claims to have little energy or patience despite sleeping over 9 hours every night. After this had persisted for several months, his wife strongly encouraged him to seek medical attention.
Diagnosis: Paul’s doctor referred him to a radiologist who specializes in positron emission tomography (PET) scans of the brain to assess brain function, as Paul was exhibiting clear symptoms of depression.
Scan: A PET scan indicated significantly lower brain activity in the majority of Paul's brain, in addition to lower glucose levels and decreased dopamine production. This provided a strong indication that Paul's recent symptoms may be due to significant decreases in his brain energy metabolism.
Treatment and Prognosis: In light of the PET scan results, Paul's doctor recommended buproprion, a norepinephrine-dopamine reuptake inhibitor, to try to increase the amount of dopamine active in his brain. Psychotherapy was also recommended. Follow-up PET scans after six months and one year indicated that both the glucose metabolic rate and amount of dopamine had increased, appearing close to normal. Paul's mood and energy levels had also improved, and he was able to participate more fully with both his family and his colleagues.
References
[edit]- ^ a b c d Blass, J.P. (2001) Brain metabolism and brain disease: Is metabolic deficiency the proximate cause of Alzheimer Dementia. J. Neuro. Res. 66, 851-856.
- ^ a b c d e Dwyer, D. (2001). Glucose Metabolism in Relation to Schizophrenia and Antipsychotic Drug Treatment. Ann of Clin Psychiatry , 13.
- ^ a b c d e f g h i j Dwyer, D. (ed.) 2002, International Review of Neurobiology: Glucose Metabolism in the Brain (vol. 51). Elsevier Science, San Diego.
- ^ a b c d Magistretti, P.J., Pellerin, L., and Martin, J. (2000) Brain Energy Metabolism: An Integrated Cellular perspective. Psychopharmacology - 4th Generation of Progress. American College of Neuropsychopharmacology.
- ^ http://en.wiki.x.io/wiki/File:Glycolysis.svg
- ^ http://en.wiki.x.io/wiki/File:Citric_acid_cycle_with_aconitate_2.svg
- ^ Cakir, T., Alsan, S., Saybasili, H., Akin, A., and Ulgen, K. (2007) Reconstruction and Flux Analysis of Coupling Between Metabolic Pathways of Astrocytes and Neurons: Application to Cerebral Hypoxia. Theor. Biol. Med. Mod. 4, doi:10.1186/1742-4682-4-48
- ^ a b Kishimoto, H. (1986). 11C-Glucose Metabolism in Manic and Depressed Patients. Psych. Res. 22(1) , 81-88.
- ^ a b Prabakaran, S. (2004). Mitochondrial dysfunction in schizophrenia:evidence for compromised brain metabolism and oxidative stress. Molec. Psych. 9 , 684-697.
- ^ a b c d http://www.cerebra.org.uk/SiteCollectionDocuments/Research%20PDF's/Brain%20scanning%20techniques.pdf
- ^ a b Seethalakshmi, R., Parkar, S., Nair, N., Adarkar, S., Pandit, A., Batra, S., Baghel, N., Moghe, S. (2006) Regional brain metabolism in schizophrenia: An FDG-PET study. Indian J. Psychiatry. 48, 149-153
- ^ a b Song, S., Kim, I., Kim, S., Kwak, K., Kim, Y. (2008) Cerebral glucose metabolism abnormalities in patients with major depressive symptoms in pre-dialytic chronic kidney disease: statistical parametric mapping analysis of F-18-FDG PET, a preliminary study. Psychiatry Clin. Neurosci. 62, 554-561.
- ^ http://www.jens-langner.de/research
- ^ a b Cannon, D., Klaver, J., Peck, S., Rallis-Voak, D., Erickson, K., Drevets, W. (2009) Dopamine type-1 receptor binding in major depressive disorder assessed using positron emission tomography and [11C]NNC-112. Neuropsychopharmacology. 5, 1277-87.
- ^ a b c Meyer, J., McNeely, H., Sagrati, S., Boovariwala, A., Martin, K., Verhoeff, N., Wilson, A., Houle, S. (2006) Elevated putamen D2 receptor binding potential in major depression with motor retardation: an [11C]raclopride positron emission tomography study. Am. J. Psychiatry. 163, 1594-1602
- ^ a b Farde, L., Wiesel, F., Stone-Elander, S., Halldin, C., Nordstrom, A., Hall, H., Sedvall, G. (1990) D2 dopamine receptors in neuroleptic-naive schizophrenic patients. A positron emission tomography study with [11C]raclopride. Arch. Gen. Psychiatry. 47, 213-9.
- ^ a b Reith, J., Benkelfat, C., Sherwin, A. (1994) Elevated DOPA decarboxylase activity in living brain of patients with psychosis. Proc. Natl. Acad. Sci. 91, 11651–11654.
- ^ a b Dao-Castellana MH, Paillere-Martinot ML, Hantraye P, et al. (1997) Presynaptic dopaminergic function in the striatum of schizophrenic patients. Schizophr. Res. 23, 167–174.
- ^ a b c Hietala J, Syvalahti E, Vilkman H, et al. (1999) Depressive symptoms and presynaptic dopamine function in neuroleptic-naive schizophrenia. Schizophr. Res. 35, 41–50.
- ^ a b Lindstrom LH, Gefvert O, Hagberg G, et al. (1999) Increased dopamine synthesis rate in medial prefrontal cortex and striatum in schizophrenia indicated by L-(beta-11C) DOPA and PET. Biol. Psychiatry. 46, 681 – 688.
- ^ a b Newberg, A., Amsterdam, J., Wintering, N., Ploessl, K., Swanson, R., Shults, J., Alavi, A. (2005) 123I-ADAM Binding to serotonin transporters in patients with major depression and healthy controls: a preliminary study. J. Nucl. Med. 46, 973-977.
- ^ Lewine, R., Risch, S., Risby, E., Stipetic, M., Jewart, R., Eccard, M., Caudie, J., Pollard, W. (1991) Lateral ventricle-brain ratio and balance between CSF-HVA and 5-HIAA in schizophrenia. Am. J. Psychiatry. 148, 1189-94.
- ^ Tank, D. W., Ogawa, S., & Ugurbil, K. (1992) Mapping the brain with MRI. Brain Imaging, 2(10), 525-528.