User:Doneuron
Neuropharmacology
[edit]Neuropharmacology is the study of how drugs affect cellular function in the nervous system. There are two main branches of neuropharmacology: behavioral and molecular. Behavioral neuropharmacology focuses on the study of how drugs affect human behavior (neuropsychopharmacology), including the study of how drug dependence and addiction affect the human brain.[1] Molecular neuropharmacology involves the study of neurons and their neurochemical interactions, with the overall goal of developing drugs that have beneficial effects on neurological function. Both of these fields are closely connected, since both are concerned with the interactions of neurotransmitters, neuropeptides, neurohormones, neuromodulators, enzymes, second messengers, co-transporters, ion channels, and receptor proteins in the central and peripheral nervous systems. Studying these interactions, researchers are developing drugs to treat many different neurological disorders, including pain, neurodegenerative diseases such as Parkinson's disease and Alzheimer's disease, psychological disorders, addiction, and many others.
Neuropharmacology did not appear in the scientific field until, in the early part of the 20th century, scientists were able to figure out a basic understanding of the nervous system and how nerves communicate between one another. Before this discovery, there were drugs, however, that had been found that demonstrated some type of influence on the nervous system. In the 1930’s, French scientists began working with a compound called phenothiazine in the hope of synthesizing a drug that would be able to combat malaria. Though this drug showed very little hope in the use against malaria infected individuals, it was found to have sedative effects along with what appeared to be beneficial effects toward patients with Parkinson’s disease. This black box method, where an investigator would administer a drug and examine the response without knowing how to relate drug action to patient response, was the main approach to this field, until, in the late 1940s and early 1950s, scientists were able to identify specific neurotransmitters, such as norepinephrine (involved in the constriction of blood vessels and the increase in heart rate and blood pressure), dopamine (the chemical whose shortage is involved in Parkinson’s disease), and serotonin (soon to be recognized as deeply connected to depression). In the 1950s, scientists also became better able to measure levels of specific neurochemicals in the body and thus correlate these levels with behavior.[2] The invention of the voltage clamp in 1949 allowed for the study of ion channels and the nerve action potential. These two major events in neuropharmacology history now allowed scientists to not only study how communication is transferred from one neuron to another, but also the specifics on how a neuron propagates this information through itself.
Psychopharmacology
[edit]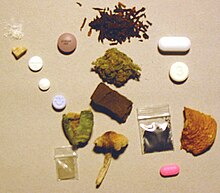
Psychopharmacology (from Greek ψῡχή, psȳkhē, "breath, life, soul"; φάρμακον, pharmakon, "drug"; and -λογία, -logia) is the study of drug-induced changes in mood, sensation, thinking, and behavior.[3]
The field of psychopharmacology studies a wide range of substances with various types of psychoactive properties. The professional and commercial fields of pharmacology and psychopharmacology do not mainly focus on psychedelic or recreational drugs, as the majority of studies are conducted for the development, study, and use of drugs for the modification of behavior and the alleviation of symptoms, particularly in the treatment of mental disorders (psychiatric medication). While studies are conducted on all psychoactives by both fields, psychopharmacology focuses primarily on the psychoactive and chemical interactions with the brain.
Psychoactive drugs may originate from natural sources such as plants and animals, or from artificial sources such as chemical synthesis in the laboratory. These drugs interact with particular target sites or receptors found in the nervous system to induce widespread changes in physiological or psychological functions. The specific interaction between drugs and their receptors is referred to as "drug action", and the widespread changes in physiological or psychological function is referred to as "drug effect". The use of psychoactive drugs predates recorded history. Hunter-gatherer societies tended to favor psychedelics, dissociatives and deliriants, and today their use can still be observed in many surviving tribal cultures. The exact drug used depends on what the particular ecosystem a given tribe lives in can support, and are typically found growing wild. Such drugs include various psychedelic mushrooms and cacti, along with many other plants. These societies generally attach spiritual significance to such drug use, and often incorporate it into their religious practices.

The common muscimol-bearing mushroom Amanita muscaria, also known as the "Fly Agaric", is frequently regarded as one of the first used psychoactive drugs, it is suspected to be the primary or active ingredient in the sacred drug of ancient India, known as Soma.[4] There are many modern theories citing the discovery of its psychoactive properties as far back as 10,000 BCE.
With the dawn of the Neolithic and the proliferation of agriculture, new psychoactives came into use as a natural by-product of farming. Among them were opium, cannabis, and alcohol derived from the fermentation of cereals and fruits. Most societies began developing herblores, lists of herbs which were good for treating various physical and mental ailments. For example, St. John's Wort was traditionally prescribed in parts of Europe for depression (in addition to use as a general-purpose tea), and Chinese medicine developed elaborate lists of herbs and preparations.
With the scientific revolution in Europe and the United States, the use of traditional herbal remedies fell out of favor with the mainstream medical establishment, although a few people continued to use and maintain knowledge of traditional European herblore. In the early 20th century, scientists began reassessing this rejection of traditional herbs in medicine. A number of important psychiatric drugs have been developed as a by-product of the analysis of organic compounds present in traditional herbal remedies. In the latter half of the 20th century, research into new psychopharmacologic drugs exploded, with many new drugs being discovered, created, and tested. Many once-popular drugs are now out of favor, and there are fashions in psychiatric drugs, as with any other kind of drug.
Only since the 1950s has the use of psychiatric drugs to restore mental health, or at least limit aberrant behavior, been a part of medical therapeutics, when a number of new classes of pharmacological agents were discovered, notably tranquillizers (e.g., chlorpromazine, reserpine, and other milder agents) and antidepressants (including the highly effective group known as tricyclic antidepressants). Additionally, psychedelic drugs (LSD and psilocybin) and empathogens (MDMA) were popularized among many psychiatrists for a certain time as very helpful tools to assist psychotherapy. Lithium is widely used to allay the symptoms of affective disorders and especially to prevent recurrences of both the manic and the depressed episodes in manic-depressive individuals. The many commercially marketed antipsychotic agents (including thiothixene, chlorpromazine, haloperidol, and thioridazine) all share the common property of blocking the dopamine receptors in the brain. (Dopamine acts to help transmit nerve impulses in the brain.) Since scientists have found a direct relationship between dopamine blockage and reduction of schizophrenic symptoms, many believe that schizophrenia may be related to excess dopamine.[5]
These drugs contrast sharply with the hypnotic and sedative drugs that formerly were in use and that clouded the patient's consciousness and impaired his/her motor and perceptual abilities. The antipsychotic drugs can allay the symptoms of anxiety and reduce agitation, delusions, and hallucinations, and the antidepressants lift spirits and quell suicidal impulses. The heavy prescription use of drugs to reduce agitation and quell anxiety has led, however, to what many psychiatrists consider an overuse of such medications.[6] An overdose of a tranquilizer may cause loss of muscular coordination and slowing of reflexes, and prolonged use can lead to addiction. Toxic side effects such as jaundice, psychoses, dependency, or a reaction similar to Parkinson's disease may develop. The drugs may produce other minor symptoms (e.g., heart palpitations, rapid pulse, sweating) because of their action on the autonomic nervous system.
Pharmacogenetics
[edit]The terms pharmacogenomics and pharmacogenetics tend to be used interchangeably, and a precise, consensus definition of either remains elusive. Pharmacogenetics is generally regarded as the study or clinical testing of genetic variation that gives rise to differing response to drugs, while pharmacogenomics is the broader application of genomic technologies to new drug discovery and further characterization of older drugs.
Much of current clinical interest is at the level of pharmacogenetics, involving variation in genes involved in, drug metabolism with a particular emphasis on improving drug safety. The wider use of pharmacogenetic testing is viewed by many as an outstanding opportunity to improve prescribing safety and efficacy. Driving this trend are the 106,000 deaths and 2.2 Million serious events caused by adverse drug reactions in the US each year (Lazarou 1998). As such ADRs are responsible for 5-7% of hospital admissions in the US and Europe, lead to the withdrawal of 4% of new medicines and cost society an amount equal to the costs of drug treatment (Ingelman-Sundberg 2005). Comparisons of the list of drugs most commonly implicated in adverse drug reactions with the list of metabolizing enzymes with known polymorphisms found that drugs commonly involved in adverse drug reactions were also those that were metabolized by enzymes with known polymorphisms (see Phillips, 2001). The decision to use pharmacogenetic techniques is influenced by the relative costs of genotyping technologies and the cost of providing a treatment to a patient with an incompatible genotype.
The first observations of genetic variation in drug response date from the 1950s, involving the muscle relaxant suxamethonium chloride, and drugs metabolized by N-acetyltransferase. One in 3500 Caucasians has less efficient variant of the enzyme (butyrylcholinesterase) that metabolizes suxamethonium chloride.[7] As a consequence, the drug’s effect is prolonged, with slower recovery from surgical paralysis. Variation in the N-acetyltransferase gene divides people into “slow acetylators” and “fast acetylators”, with very different half-lives and blood concentrations of such important drugs as isoniazid (antituberculosis) and procainamide (antiarrhythmic). As part of the inborn system for clearing the body of xenobiotics, the cytochrome P450 oxidases (CYPs) are heavily involved in drug metabolism, and genetic variations in CYPs affect large populations. One member of the CYP superfamily, CYP2D6, now has over 75 known allelic variations, some of which lead to no activity, and some to enhanced activity. An estimated 29% of people in parts of East Africa may have multiple copies of the gene, and will therefore not be adequately treated with standard doses of drugs such as the painkiller codeine (which is activated by the enzyme).
One of the earliest tests for a genetic variation resulting in a clinically important consequence was on the enzyme thiopurine methyltransferase (TPMT). TPMT metabolizes 6-mercaptopurine and azathioprine, two thiopurine drugs used in a range of indications, from childhood leukemia to autoimmune diseases. In people with a deficiency in TPMT activity, thiopurine metabolism must proceed by other pathways, one of which leads to the active thiopurine metabolite that is toxic to the bone marrow at high concentrations. Deficiency of TPMT affects a small proportion of people, though seriously. One in 300 people have two variant alleles and lack TPMT activity; these people need only 6-10% of the standard dose of the drug, and, if treated with the full dose, are at risk of severe bone marrow suppression. For them, genotype predicts clinical outcome, a prerequisite for an effective pharmacogenetic test. In 85-90% of affected people, this deficiency results from one of three common variant alleles. Around 10% of people are heterozygous - they carry one variant allele - and produce a reduced quantity of functional enzyme. Overall, they are at greater risk of adverse effects, although as individuals their genotype is not necessarily predictive of their clinical outcome, which makes the interpretation of a clinical test difficult. Recent research suggests that patients who are heterozygous may have a better response to treatment, which raises whether people who have two wild-type alleles could tolerate a higher therapeutic dose. The US Food and Drug Administration (FDA) have recently deliberated the inclusion of a recommendation for testing for TPMT deficiency to the prescribing information for 6-mercaptopurine and azathioprine. Hitherto the information has carried the warning that inherited deficiency of the enzyme could increase the risk of severe bone marrow suppression. Now it will carry the recommendation that people who develop bone marrow suppression while receiving 6-mercaptopurine or azathioprine be tested for TPMT deficiency.
Clinical Neuropharmacology
[edit]Clinical pharmacology is the science of drugs and their clinical use. It is underpinned by the basic science of pharmacology, with added focus on the application of pharmacological principles and methods in the real world. It has a broad scope, from the discovery of new target molecules, to the effects of drug usage in whole populations.
A recent breakthrough in pharmacogenetics identified a polymorphism near a human interferon gene that is predictive of the effectiveness of an artificial interferon treatment for Hepatitis C. For genotype 1 hepatitis C treated with Pegylated_interferon-alpha-2a or Pegylated_interferon-alpha-2b (brand names Pegasys or PEG-Intron) combined with ribavirin, it has been shown that genetic polymorphisms near the human IL28B gene, encoding interferon lambda 3, are associated with significant differences in response to the treatment. This finding, originally reported in Nature,[8] showed that genotype 1 hepatitis C patients carrying certain genetic variant alleles near the IL28B gene are more possibly to achieve sustained sustained virological response after the treatment than others. Later report from Nature[9] demonstrated that the same genetic variants are also associated with the natural clearance of the genotype 1 hepatitis C virus.
Clinical pharmacology connects the gap between medical practice and laboratory science. The main objective is to promote the safety of prescription, maximise the drug effects and minimise the side effects. It is important that there be association with pharmacists skilled in areas of drug information, medication safety and other aspects of pharmacy practice related to clinical pharmacology.
Clinical pharmacologists usually have a rigorous medical and scientific training which enables them to evaluate evidence and produce new data through well designed studies. Clinical pharmacologists must have access to enough outpatients for clinical care, teaching and education, and research as well be supervised by medical specialists. Their responsibilities to patients include, but are not limited to analyzing adverse drug effects, therapeutics, and toxicology including reproductive toxicology, cardiovascular risks, perioperative drug management and psychopharmacology.
In addition, the application of genetic, biochemical, or virotherapuetical techniques has led to a clear appreciation of the mechanisms involved in drug action.
Pharmacodynamics
[edit]Pharmacodynamics is the study of the physiological effects of drugs on the body or on microorganisms or parasites within or on the body and the mechanisms of drug action and the relationship between drug concentration and effect.[10] One dominant example is drug-receptor interactions as modeled by
where L=ligand (drug), R=receptor (attachment site), reaction dynamics that can be studied mathematically through tools such as free energy maps. Pharmacodynamics is often summarized as the study of what a drug does to the body, whereas pharmacokinetics is the study of what the body does to a drug. Pharmacodynamics is sometimes abbreviated as "PD", and when referred to in conjunction with pharmacokinetics can be referred to as "PK".
Effects on the body
[edit]The majority of drugs either (a) mimic or inhibit normal physiological/biochemical processes or inhibit pathological processes in animals or (b) inhibit vital processes of endo- or ectoparasites and microbial organisms.
There are 5 main drug actions:
- depressing
- stimulating
- destroying cells (cytotoxicity)
- irritation
- replacing substances
Desired activity
[edit]The desired activity of a drug is mainly due to one of the following:
- Cellular membrane disruption
General anesthetics were once thought to work by disordering the neural membranes, thereby altering the Na+ influx.
Antacids and chelating agents combine chemically in the body.
- Interaction with enzyme proteins
Enzyme-substrate binding is a way to alter the production or metabolism of key endogenous chemicals, for example aspirin irreversibly inhibits the enzyme prostaglandin synthetase (cyclooxygenase) thereby preventing inflammatory response.
- Interaction with structural proteins
Colchicine, a drug for gout, interferes with the function of the structural protein tubulin, while Digitalis, a drug still used in heart failure, inhibits the activity of the carrier molecule, Na-K-ATPase pump.
- Interaction with carrier proteins
Cocaine and antidepressants, like Prozac, block the monoamine transporters that clear monoamines from the synapse.
- Interaction with ion channels
Nicotine is an example of an agonist that opens the nicotinic acetylcholine receptor/channel complex
- Ligand binding to Hormone receptors,Neuromodulator receptors,Neurotransmitter receptors
The widest class of drugs act as ligands which bind to receptors which determine cellular effects. Upon drug binding, receptors can elicit their normal action (agonist), blocked action (antagonist), or even action opposite to normal (inverse agonist).
In principle, a pharmacologist would aim for a target plasma concentration of the drug for a desired level of response. In reality, there are many factors affecting this goal. Pharmacokinetic factors determine peak concentrations, and concentrations cannot be maintained with absolute consistency because of metabolic breakdown and excretory clearance. Genetic factors may exist which would alter metabolism or drug action itself, and a patient's immediate status may also affect indicated dosage.
Undesirable effects
[edit]Undesirable effects of a drug include:
- Increased probability of cell mutation (carcinogenic activity)
- A multitude of simultaneous assorted actions which may be deleterious
- Interaction (additive, multiplicative, or metabolic)
- Induced physiological damage, or abnormal chronic conditions
Therapeutic window
[edit]The therapeutic window is the amount of a medication between the amount that gives an effect (effective dose) and the amount that gives more adverse effects than desired effects. For instance, medication with a small pharmaceutical window must be administered with care and control, e.g. by frequently measuring blood concentration of the drug, since it easily loses effects or gives adverse effects.
Receptor binding
[edit]The binding of ligands (drug) to receptors is governed by the law of mass action which relates the large-scale status to the rate of numerous molecular processes. The rates of formation and un-formation can be used to determine the equilibrium concentration of bound receptors. The equilibrium dissociation constant is defined by:
where L=ligand, R=receptor, square brackets [] denote concentration. The fraction of bound receptors is found as (1+[R]/[L·R])-1 , which can then be expressed using Kd as,
This expression is one way to consider the effect of a drug, in which the response is related to the fraction of bound receptors. The fraction of bound receptors is known as occupancy. The relationship between occupancy and pharmacological response is usually non-linear. This explains the so called receptor reserve phenomenon i.e. the concentration producing 50% occupancy is typically higher than the concentration producing 50% of maximum response.
Often the response is determined as a function of log[L] to consider many orders of magnitude of concentration. However, there is no biological or physical theory which relates effects to the log of concentration. It is just convenient for graphing purposes. It is useful to note that 50% of the receptors are bound when [L]=Kd .
The graph shown represents the conc-response for two hypothetical receptor agonists, plotted in a semi-log fashion. The curve toward the left represents a higher potency (potency arrow does not indicate direction of increase) since lower concentrations are needed for a given response. The effect increases as a function of concentration.
Neurochemical Interactions
[edit]To understand the potential advances in medicine that neuropharmacology can bring, it is important to understand how human behavior and thought processes are transferred from neuron to neuron and how medications can alter the chemical foundations of these processes.
Neurons are known as excitable cells because on its surface membrane there are an abundance of proteins known as ion-channels that allow small charged particles to pass in and out of the cell. The structure of the neuron allows chemical information to be received by its dendrites, propagated through the soma (cell body) and down its axon, and eventually passing on to other neurons through its axon terminal.

These voltage-gated ion channels allow for rapid depolarization throughout the cell. This depolarization, if it reaches a certain threshold, will cause an action potential. Once the action potential reaches the axon terminal, it will cause an influx of calcium ions into the cell. The calcium ions will then cause vesicles, small packets filled with neurotransmitters, to bind to the cell membrane and release its contents into the synapse. This cell is known as the pre-synaptic neuron, and the cell that interacts with the neurotransmitters released is known as the post-synaptic neuron. Once the neurotransmitter is released into the synapse, it can either bind to receptors on the post-synaptic cell, the pre-synaptic cell can re-uptake it and save it for later transmission, or it can be broken down by enzymes in the synapse specific to that certain neurotransmitter. These three different actions are major areas where drug action can effect communication between neurons.[2]
There are two types of receptors that neurotransmitters interact with on a post-synaptic neuron. The first types of receptors are ligand-gated ion channels or LGIC’s. LGIC receptors are the fastest types of transduction from chemical signal to electrical signal. Once the neurotransmitter binds to the receptor it will cause a conformational change that will allow ions to directly flow into the cell. The second types are known as G-protein-coupled receptors or GPCR’s. These are much slower than LGIC’s due to an increase in the amount of biochemical reactions that must take place intracellularly. Once the neurotransmitter binds to the GPCR protein it causes a cascade of intracellular interactions that can lead to many different types of changes in cellular biochemistry, physiology, and gene expression. Neurotransmitter/receptor interactions in the field of neuropharmacology are extremely important because many drugs that are developed today have to do with disrupting this binding process.
Pharmacokinetics is how the drug moves through the body. This involves the body systems for handling the drug, usually divided into the following classifcation:
- Absorption
- Distribution
- Metabolism
- Elimination
- Rational Prescribing - using the right medication, at the right dose, using the right route and frequency of administration for the patient, and stopping the drug appropriately.
- Adverse Drug Effects
- Toxicology
- Drug interactions
- Drug development - usually culminating in some form of clinical trial.
Molecular Neuropharmacology
[edit]Molecular neuropharmacology involves the study of neurons and the neurochemical interactions, and neuron receptors with the goal of developing new drugs that will treat neurological disorders such as pain, neurodegenerative diseases, and psychological disorders (also known as neuropsychopharmacology).There are a few terminology words that must be defined when relating neurotransmission to receptor action.
- Agonist- this is when a molecule binds to a receptor protein and activates that receptor
- Competitive Antagonist- this is when a molecule binds to the same site on the receptor protein as the agonist, preventing activation of the receptor.
- Non-competitive Antagonist- this is when a molecule binds to a receptor protein on a different site than that of the agonist, but causes a conformational change in the protein that does not allow activation.
The following neurotransmitter/receptor interactions can be affected by synthetic compounds that act as one of the three above. Sodium/Potassium ion channels can also be manipulated throughout a neuron to induce inhibitory effects of action potentials.
GABA
[edit]The GABA neurotransmitter mediates the fast synaptic inhibition in the central nervous system. When GABA is released from its pre-synaptic cell it will bind to a receptor (most likely the GABAA receptor) that causes the post-synaptic cell to hyperpolarize (stay below its action potential threshold). This will counteract the effect of any excitatory manipulation from other neurotransmitter/receptor interactions.
This GABAA receptor contains many binding sites that allow conformational changes and are the primary target for drug development. The most common of these binding sites, benzodiazepine, allows for both agonist and antagonist effects on the receptor. A common drug, diazepam, acts as an allosteric enhancer at this binding site.[11] Another receptor for GABA, known as GABAB, can be enhanced by a molecule called baclofen. This molecule acts as an agonist, therefore activating the receptor, and is known to help control and decrease spastic movement.
Dopamine
[edit]The dopamine neurotransmitter mediates synaptic transmission by binding to five specific GPCR's. These five receptor proteins are separated into two classes due to whether the response elicits a excitatory or inhibitory response on the post-synaptic cell. There are many types of drugs, legal and illegal, that effect dopamine and its interactions in the brain. With Parkinson's disease, a disease that decreases the amount of dopamine in the brain, the dopamine precursor Levadopa is given to the patient due to the fact that dopamine cannot cross the blood-brain barrier and L-dopa can. Some dopamine agonists are also given to Parkinson's patients that have a disorder known as restless leg syndrome or RLS. Some examples of these are ropinirole and pramipexole.[12]
Psychological disorders like that of attention deficit hyperactivity disorder (ADHD) can be treated with drugs like methylphenidate (also known as Ritalin) which block the re-uptake of dopamine by the pre-synaptic cell, thereby providing an increase of dopamine left in the synaptic gap. This increase in synaptic dopamine will increase binding to receptors of the post-synaptic cell. This same process is also used by other illegal stimulant drugs like that of cocaine and amphetamines.
Serotonin
[edit]The serotonin neurotransmitter has the ability to mediate synaptic transmission through either GPCR's or LGIC receptors. Depending on what part of the brain region serotonin is being acted upon, will depend on whether the output is either increasing or decreasing post-synaptic responses. The most popular and widely used drugs in the regulation of serotonin during depression are known as SSRI's or selective serotonin reuptake inhibitors. These drugs inhibit the transport of serotonin back into the pre-synaptic neuron, leaving more serotonin in the synaptic gap to be used.
Before the discovery of SSRI's, there were also very many drugs that inhibited the enzyme that broke down serotonin. MAOI's or monoamine oxidase inhibitors increased the amount of serotonin in the pre-synaptic cell, but had many side effects including intense migraines and high blood pressure. This was eventually linked to the drug interacting with a common chemical known as tyramine found in many types of food.[13]
Ion Channels
[edit]Ion channels located on the surface membrane of the neuron, allows for an influx of sodium ions and outward movement of potassium ions during an action potential. Selectively blocking these ion channels will decrease the likelihood of an action potential to occur. The drug riluzole is a neuroprotective drug that blocks sodium ion channels. Since these channels can not activate, there is no action potential and the neuron does not perform any transduction of chemical signals into electrical signals and the signal does not move on. This drug is used as an anesthetic along with sedative properties.
Behavioral Neuropharmacology
[edit]
One form of behavioral neuropharmacology focuses on the study of drug dependence and how drug addiction affects the human mind. (see neuropsychopharmacology for human behavior and drug development) Most research has shown that the major part of the brain that reinforces addiction through neurochemical reward is the nucleus accumbens. The image to the right shows how dopamine and serotonin are projected into this area.
Research
[edit]Parkinson's Disease
[edit]Parkinson's disease is a neurodegenerative disease described by the selective loss of dopaminergic neurons located in the substantia nigra. Today, the most commonly used drug to combat this disease is levodopa or L-DOPA. This precursor to dopamine can penetrate through the blood-brain barrier whereas the neurotransmitter dopamine cannot. There has been extensive research to determine whether L-dopa is a better treatment for Parkinson's disease rather than other dopamine agonists. Some believe that the long term use of L-dopa will compromise neuroprotection and thus eventually lead to dopaminergic cell death. Though there has been no proof, in-vivo or in-vitro, some still believe that the better long-term use of dopamine agonists be better for the patient.[14]
Alzheimer's Disease
[edit]While there are a variety of hypotheses that have been proposed for the cause of Alzheimer's disease, the knowledge of this disease is far from complete to explain, making it difficult to develop methods for treatment. In the brain of Alzheimer's patients, both neuronal nicotinic acetylcholine (nACh) receptors and NMDA receptors are known to be down-regulated. Thus four anticholinesterases have been developed and approved by the U.S. Food and Drug Administration (FDA) for the treatment in the U.S.A. However, these are not ideal drugs considering their side effects and limited effectiveness. One promising drug, nefiracetam, is being developed for the treatment of Alzheimer's and other patients with dementia, and has unique actions in potentiating the activity of both nACh receptors and NMDA receptors.[15]
Psychopharmacology Research
[edit]In psychopharmacology, researchers are interested in any substance that crosses the blood-brain barrier and thus has an effect on behavior, mood or cognition. Drugs are researched for their physicochemical properties, physical side effects, and psychological side effects. Researchers in psychopharmacology study a variety of different psychoactive substances that include alcohol, cannabinoids, club drugs, psychedelics, opiates, nicotine, caffeine, psychomotor stimulants, inhalants, and anabolic-androgenic steroids. They also study drugs used in the treatment of affective and anxiety disorders, as well as schizophrenia.
Clinical studies are often very specific, typically beginning with animal testing, and ending with human testing. In the human testing phase, there is often a group of subjects, one group is given a placebo, and the other is administered a carefully measured therapeutic dose of the drug in question. After all of the testing is completed, the drug is proposed to the concerned regulatory authority (e.g. the U.S. FDA), and is either commercially introduced to the public, introduced to the public via prescription, or deemed safe enough for over the counter sale.
Though particular drugs are prescribed for specific symptoms or syndromes, they are usually not specific to the treatment of any single mental disorder. Because of their ability to modify the behavior of even the most disturbed patients, the antipsychotic, antianxiety, and antidepressant agents have greatly affected the management of the hospitalized mentally ill, enabling hospital staff to devote more of their attention to therapeutic efforts and enabling many patients to lead relatively normal lives outside of the hospital. A somewhat controversial application of psychopharmacology is "cosmetic psychiatry" Persons who do not meet criteria for any psychiatric disorder are nevertheless prescribed psychotropic medication. The antidepressant Wellbutrin is then prescribed to increase perceived energy levels and assertiveness while diminishing the need for sleep. The antihypertensive compound Inderal is sometimes chosen to eliminate the discomfort of day-to-day "normal" anxiety . Prozac in nondepressed people can produce a feeling of generalized well-being. Mirapex, a treatment for restless leg syndrome, can dramatically increase libido in women. These and other off-label life-style applications of medications are not uncommon. Although occasionally reported in the medical literature no guidelines for such usage have been developed. [16]
References
[edit]- ^ Everitt, B. J., & Robbins, T. W. (2005). "Neural systems of reinforcement for drug addiction: from actions to habits to compulsion". Nature Neuroscience. 8 (11): 1481–1489.
{{cite journal}}
: CS1 maint: multiple names: authors list (link) - ^ a b Wrobel, S. (2007). "Science, serotonin, and sadness: the biology of antidepressants - A series for the public. [Editorial Material]". Faseb Journal. 21 (13): 3404–3417.
- ^ Meyer, J. S. and Quenzer, L. S. (2004). Psychopharmacology: Drugs, the Brain and Behavior. Sinauer Associates. ISBN 0-87893-534-7.
- ^ Mike Crowley (1996). "When the Gods Drank Urine". Fortean Studies, vol. III.
- ^ Horrobin DF (1979). "Schizophrenia: Reconciliation of the dopamine, prostaglandin, and opioid concepts and the role of the pineal". Lancet. 313 (8115): 529. doi:10.1016/S0140-6736(79)90948-6. PMID 85110.
{{cite journal}}
:|access-date=
requires|url=
(help); Unknown parameter|month=
ignored (help) - ^ Anne Collins Abrams, Carol Barnett Lammon, Sandra Smith Pennington (2007). Clinical Drug Therapy: Rationales for Nursing Practice. Philadelphia: Lippincott Williams & Wilkins. ISBN 0781762634.
{{cite book}}
: CS1 maint: multiple names: authors list (link) - ^ Gardiner SJ, Begg EJ (2006). "Pharmacogenetics, drug-metabolizing enzymes, and clinical practice". Pharmacol. Rev. 58 (3): 521–90. doi:10.1124/pr.58.3.6. PMID 16968950.
{{cite journal}}
: Unknown parameter|month=
ignored (help) - ^ Ge D, Fellay J, Thompson AJ, Simon JS, Shianna KV, Urban TJ, Heinzen EL, Qiu P, Bertelsen AH, Muir AJ, Sulkowski M, McHutchison JG, Goldstein DB (2009). "Genetic variation in IL28B predicts hepatitis C treatment-induced viral clearance". Nature. 461 (7262): 399–401. doi:10.1038/nature08309. PMID 19684573.
{{cite journal}}
: Unknown parameter|month=
ignored (help)CS1 maint: multiple names: authors list (link) - ^ Thomas DL, Thio CL, Martin MP, Qi Y, Ge D, O'Huigin C, Kidd J, Kidd K, Khakoo SI, Alexander G, Goedert JJ, Kirk GD, Donfield SM, Rosen HR, Tobler LH, Busch MP, McHutchison JG, Goldstein DB, Carrington M (2009). "Genetic variation in IL28B and spontaneous clearance of hepatitis C virus". Nature. 461 (7265): 798–801. doi:10.1038/nature08463. PMID 19759533.
{{cite journal}}
: Unknown parameter|month=
ignored (help)CS1 maint: multiple names: authors list (link) - ^ Lees P, Cunningham FM, Elliott J (2004). "Principles of pharmacodynamics and their applications in veterinary pharmacology". J. Vet. Pharmacol. Ther. 27 (6): 397–414. doi:10.1111/j.1365-2885.2004.00620.x. PMID 15601436.
{{cite journal}}
: CS1 maint: multiple names: authors list (link) - ^ Sigel, E. (2002). "Mapping of the benzodiazepine recognition site of GABAA receptors". Current Topics in Medicinal Chemistry. 2 (8): 833–839.
- ^ Winkelman, J.W.; Allen, R.P.; Tenzer, P.; and Hening, W. (2007). "Restless legs syndrome: Nonpharmacologic and pharmacologic treatments". Geriatrics. 62 (10): 13–16.
{{cite journal}}
: CS1 maint: multiple names: authors list (link) - ^ Lopez-Munoz, F., & Alamo, C. (2009). "Monoaminergic Neurotransmission: The History of the Discovery of Antidepressants from 1950s Until Today. [Review]". Current Pharmaceutical Design. 15 (14): 1563–1586.
{{cite journal}}
: CS1 maint: multiple names: authors list (link) - ^ Shin, J. Y., Park, H. J., Ahn, Y. H., & Lee, P. H. (2009). "Neuroprotective effect of l-dopa on dopaminergic neurons is comparable to pramipexol in MPTP-treated animal model of Parkinson's disease: a direct comparison study". Journal of Neurochemistry. 111 (4): 1042–1050.
{{cite journal}}
: CS1 maint: multiple names: authors list (link) - ^ Narahashi, T., Marszalec, W., Moriguchi, S., Yeh, J. Z., & Zhao, X. L. (2003). "Unique mechanism of action of Alzheimer's drugs on brain nicotinic acetylcholine receptors and NMDA receptors". Life Sciences. 74 (2–3): 281–291.
{{cite journal}}
: CS1 maint: multiple names: authors list (link) - ^ AJ Giannini.The case for cosmetic psychiatry: Treatment without diagnosis. Psychiatric Times. 21(7):1-2,2004